Product Name: | Sarin. | Synonyms: | Sarin.;SARINTYPEII;O-ISOPROPYLMETHYLPHOSPHONOFLUORIDATE;AGENTGB;SARIN-I;ISOPROPYLMETHYLPHOSPHONOFLUORIDATE;SARINTYPEI;SARIN-II | CAS: | 107-44-8 | MF: | C4H10FO2P | MW: | 140.09 | EINECS: | | Product Categories: | | Mol File: | 107-44-8.mol | 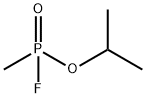 |
| Sarin. Chemical Properties |
Melting point | -57° | Boiling point | bp760 147°; bp16 56° | density | d420 1.10 | form | liquid | Stability: | Stability | EPA Substance Registry System | Sarin (107-44-8) |
| Sarin. Usage And Synthesis |
Description | Sarin, also known as "nerve agent Gas B" or GB, is an organophosphorus compound,
a colourless and odourless liquid, and a potent inhibitor of the cholinesterase enzyme.
Sarin reacts with steam or water to produce toxic and corrosive gases. Sarin is incompatible
with tin, magnesium, cadmium-plated steel, and some aluminium and reacts
with copper, brass, and lead. Sarin is the most volatile of the nerve agents, which
means that it can easily and quickly evaporate from a liquid into a vapour and spread
into the environment. People can be exposed to the vapour even if they do not come
in contact with the liquid form of sarin. | Chemical Properties | Sarin (GB), a nerve agent, is one of the most
toxic of the known chemical warfare agents. Exposure to
sarin can cause death in minutes. A fraction of an ounce
(1 to 10 mL) of GB on the skin can be fatal. GB is an
odorless, colorless, tasteless, nonflammable liquid @ 15 ℃
and 1 atm. GB has no warning properties, especially when
pure, and it can take away your sense of smell. | Uses | Sarin is a synthetic OP compound developed to be used in
chemical warfare and terrorism. | Uses | Chemical warfare agent. | Definition | ChEBI: Isopropyl methylphosphonofluoridate is a phosphinic ester that is the isopropyl ester of methylphosphonofluoridic acid. It is a phosphinic ester and a fluorine molecular entity. | General Description | A colorless, odorless liquid. Almost no odor in pure state. Used as a quick-acting military chemical nerve agent. Chemical warfare agent. | Air & Water Reactions | Hydrolyzed by water, rapidly hydrolyzed by dilute aqueous sodium hydroxide. Water alone removes Fluoride atom producing nontoxic acid [Merck 11th ed. 1989]. | Reactivity Profile | Acidic conditions produce hydrogen fluoride; alkaline conditions produce isopropyl alcohol and polymers. When heated to decomposition or reacted with steam, Sarin. emits very toxic fumes of fluorides and oxides of phosphorus. Slightly corrosive to steel. Hydrolyzed by water [EPA, 1998]. | Health Hazard | Sarin is one of the most toxic compounds ever synthesized. It is a very powerfulinhibitor of acetylcholinesterase enzyme. It exhibits systemic toxicity to the central nervous system. The liquid can be absorbed readily through the skin. One drop so absorbed can be fatal to humans (Manahan 1989). It is extremely toxic by inhalation and oral intake. The toxic symptoms are similar to those of the insecticide parathion but more severe than the latter. In humans the toxic effects from sarin may be manifested at an oral dose as low as 0.002–0.005 mg/kg with symptoms of nausea, vomiting, muscle weakness, bronchiolar constriction, and asthma. A lethal dose may be as low as 0.01 mg/kg. A LC50 value from 10-minute exposure may be 100-150 mg/m3 for most animals; and the oral LD50 value for rats should be within the range of 0.5 mg/kg. Mioduszewski et al. (2001) estimated the probability of sarin vapor-induced lethality in Sprague-Dawley rats exposed to various combinations of exposure concentration and duration in a whole-body dynamic chamber. In this study, the onset of clinical signs and changes in blood cholinesterase activity were measured with each exposure. Miosis was the initial clinical sign observed at the start of the exposure. The authors have reported that the interaction of LC50× time (LCT 50) values increased with each exposure duration and found poor correlation of cholinesterase inhibition to exposure conditions or to the severity of clinical signs. Bide and Risk (2004) studied the inhalation toxicity of sarin in mice from longer exposures to low-level concentrations in an effort to establish toxicity estimates for humans. The exposure period ranged from 20 minutes to 12 hours in their study. The LCT 50 values for 20-, 60-, 180-, 360-, and 720minute exposures were 430, 540, 900, 1210, and 2210 mgDmin/m3, respectively, corresponding to LC50 values 21.5, 9.0, 5.0, 3.4, and 3.1 mg/m3, respectively. The authors reported their data did not fit Haber’s rule LCT50=CT in predicting the toxicity for longer exposures. The LC50 and LCT 50 values for 3-12 h were progressively higher (toxicity lower) than those predicted either by Haber’s rule or the “toxic load model”. The 20- and 60-minute data fit the “toxic load model” established previously from historical data for 0.17-30 min sarin exposures in mice. In a study on the toxicity of sarin, soman, and tabun in brain acetylcholinesterase activity in mice, Tripathi and Dewey (1989) observed differential potencies between lethality and inhibition of acetylcholinesterase. These investigators conclude that there were more factors involved than simply the inhibition of acetylcholinesterase within the brain, causing lethality of these nerve agents. An LD50 value by intravenous administration for sarin was reported as 0.109 mg/kg. The acute toxicity of sarin based on behavioral test models indicated that certain species, such as marmosets, showed greater sensitivity to the lethal action of sarin than rodents and rabbits (D’Mello and Duffey 1985). Doses in the range 33–55% LD50 produced 88% or more inhibition of erythrocyte acetylcholinesterase and disrupted the performance of a foodreinforced visually guided reaching response. Acute neurotoxic investigation using perfused canine brain indicated that 400 μg of sarin caused seizure in 5.6 minutes after stimulation of cerebral metabolic activity (Drewes and Singh 1987). Inhalation of sarin and soman in amounts of 30 and 13.14 μg/kg, respectively, caused irregular pulse rate, development of apnea, and a significant decrease in mean blood pressure in baboons (Anzueto et al. 1990). These effects were reversed with atropine. Yokoyama et al. (1998) have evaluated the chronic neurobehavioral effects related to post-traumatic stress disorder induced by exposure to sarin in male and female patients in the Tokyo subway incident in 1995. Nakajima et al. (1998) conducted an epidemiological study and discussed the muscarinic and nicotinic signs in the victims of sarin poisoning in Matsumoto City, Japan, in 1994. Goldman et al. (1987) evaluated mutagenic potential of sarin and soman in vitro and in vivo. Both these nerve agents gave no significant evidence of mutagenicity. | Health Hazard | Extremely toxic; lethal dose in humans may be as low as 0.01 mg/kg. Extremely active cholinesterase inhibitor. Toxic effects similar to, but more severe than those of parathion. Death within 15 minutes after fatal dose is absorbed. | Fire Hazard | Non-flammable. Acidic conditions produce hydrogen fluoride; alkaline conditions produce isopropyl alcohol and polymers. When heated to decomposition or reacted with steam, Sarin. emits very toxic fumes of fluorides and oxides of phosphorus. Slightly corrosive to steel. Hydrolyzed by water. | Potential Exposure | GB is used as a quick-acting chemical
warfare nerve agent; nerve gas. Both the liquid and the
vapor can kill you. Very small amounts can hurt you in one
minute or less, and can quickly lead to death. A single
drop, if vaporized, can kill everyone in a room ! Sarin is
26 times more deadly than cyanide gas and 20 times more
deadly than Potassium cyanide. | Shipping | UN2810 Toxic liquids, organic, n.o.s., Hazard
Class: 6.1; Labels: 6.1-Poison Inhalation Hazard, Technical
Name Required. Driver shall be given full and complete
information regarding shipment and conditions in case of
emergency. AR 50-6 deals specifically with the shipment
of chemical agents. Shipments of agent will be escorted in
accordance with AR 740-32. Passenger aircraft/rail:
FORBIDDEN; Cargo aircraft only: FORBIDDEN. The
packaging and shipping of samples are subject to strict
regulations established by the Department of
Transportation (DOT), Center for Disease Control, United
States Postal Service, OSHA, and International Air
Transport Association). Military driver shall be given full
and complete information regarding shipment and condi-
tions in case of emergency. AR 50-6 deals specifically with
the shipment of chemical agents. Shipments of agent will
be escorted in accordance with AR 740-32. | Toxicity evaluation | Sarin and other nerve agents are irreversible cholinesterase
inhibitors. Clinical effects of exposure result primarily from
inhibition of acetylcholinesterase (AChE) and butyrylcholinesterase
(BuChE). Normally, AChE is responsible for
degradation of the neurotransmitter acetylcholine in both the
peripheral and central nervous systems (CNS). Acetylcholine
stimulates contraction of skeletal muscles, and hydrolysis by
AChE prevents continual overstimulation of the acetylcholine
receptors. Inhibition of AChE blocks degradation of acetylcholine,
resulting in an accumulation of acetylcholine and
cholinergic overstimulation of the target tissues. AChE inhibition
can have muscarinic, nicotinic, and CNS effects, resulting
in a variety of symptoms, including involuntary muscle
contractions, seizures, and increased fluid secretion (e.g., tears,
saliva). The cause of death is typically respiratory dysfunction
resulting from paralysis of the respiratory muscles, bronchoconstriction,
buildup of pulmonary secretions, and depression
of the brain’s respiratory center.
Cholinesterases in the blood are often used to approximate
AChE tissue levels following exposure to a nerve agent. Red
blood cell cholinesterase (RBC-ChE) is found on erythrocytes
and BuChE in blood plasma. Affinities of cholinesterase
inhibitors for BuChE or RBC-ChE vary. The turnover rate for
RBC-ChE enzyme activity is the same as that for red blood cell
turnover at w1% per day. Tissue AChE and plasma BuChE
activities return with synthesis of new enzymes, the rate of
which differs between plasma and tissues as well as between
different tissues.
Binding of nerve agents to AChE is generally considered to
be irreversible unless removed by therapy. Oximes are used as
therapeutics to reactivate the enzyme prior to ‘aging’ or the
point at which the agent–enzyme complex is covalently linked
and the enzyme cannot be reactivated. Spontaneous reactivation
in the absence of oximes is possible but is unlikely to
occur at a rate sufficient to be clinically important. The time
required for 50% of the enzyme to become resistant to reactivation
varies by nerve agent. For sarin, the t1/2 for AChE
is w3 h and for RBC-ChE w5 h.
It is known that OP cholinesterase inhibitors exert their
toxic effects through mechanisms other than AChE inhibition.
A 1978 study by Van Meter, Karczamar, and Fiscus showed that
administering a second dose of sarin to rabbits still induced
seizures even though the brain AChE was already inhibited by
the previous dose of sarin. Further, pretreatment protection of
AChE with physostigmine still resulted in death after high-dose
treatment with nerve agent. Finally, it has been shown that
mice lacking AChE are actually more sensitive to OP poisoning
(including sarin) than wild type mice, supporting that fact that
inhibition of AChE is not the only cause of toxic effects.
One of the noncholinergic effects resultant from treatment
with OP nerve agents is changes in the levels of neurotransmitters
other than acetylcholine. These include g-amino-butyric
acid (GABA), dopamine, serotonin, and norepinephrine. While
the exact mechanism by which nerve agent exposure alters
the levels of these neurotransmitters is not known, it is thought
that these changes may be due to a compensatory mechanism
in response to overstimulation of the cholinergic system, direct
action of the OP on the proteins responsible for noncholinergic
neurotransmission, or perhaps both. Nerve agents have also
been shown to inhibit a family of enzymes called serine esterases,
which play an important role in the metabolism and
persistence of neuropeptides such as endorphins and enkephalins.
Neuroinflammation as a result of nerve agent exposure
is another possible mechanism for noncholinergic toxicity
effects. OPs have also been shown to have direct toxic effects on
cells via induction of cellular oxidative stress and mitochondrial
dysfunction. | Incompatibilities | Attacks tin, magnesium, cadmium plated
steel; and some aluminums. GB decomposes tin, magne-
sium, cadmium-plated steel, and aluminum. Slightly corro-
sive to brass, copper, and lead. No attack on 1020 steel,
Inconel, and K-Monel. Hydrolyzed by water. In acid condi-
tions, GB hydrolyzes, forming hydrofluoric acid (HF).
Rapidly hydrolyzed by dilute aqueous sodium hydroxide
(NaOH), or sodium carbonate, forming relatively nontoxic
products of polymers and isopropyl alcohol. Contact with
metals may evolve flammable hydrogen gas. | Waste Disposal | It is dissolved in a combustible solvent and burned in a chemical incinerator equipped with an afterburner and scrubber. Sarin may also be destroyed by the Shultz process of molten metal reduction (Shultz 1987). Molten aluminum, aluminum alloys, recovered scrap metal, or eutectic melts may be used at 780-1000°C (1436-1832°F). Sarin is reduced to phosphorus, alkenes, and hydrogen. The hydrocarbon products may be used in preheating the feed. Worley (1989) reported decomposition of sarin,soman,VX,andother chemicalwarfare agentsbyoxidizingwith1,3-dibromo-4,4,5,5tetramethyl-2-imidazolidinoneorotherN,N0dihalo-2-imidazolidinone. The reaction is carriedoutinanaqueousemulsioncontaining tetrachloroethylene or a similar organic solvent. Sarin and other nerve agents may be removed from cleaning organic solvents (trichlorotrifluoroethane and its mixtures) by such adsorbents as Fuller’s earth, activated alumina, silica gel, and silica gel impregnated with a metal salt (Fowler and McIlvaine 1989). Hydrolysis with water or dilute alkaliesshould yieldproductsof low toxicity. . |
| Sarin. Preparation Products And Raw materials |
|